Lessons From The Past And Present For Controlling Covid-19: Polio
(Posted on Monday, March 8, 2021)
This article is the first in an ongoing series about what we can learn from successful efforts to vaccinate against other diseases and apply to interventions against Covid-19.
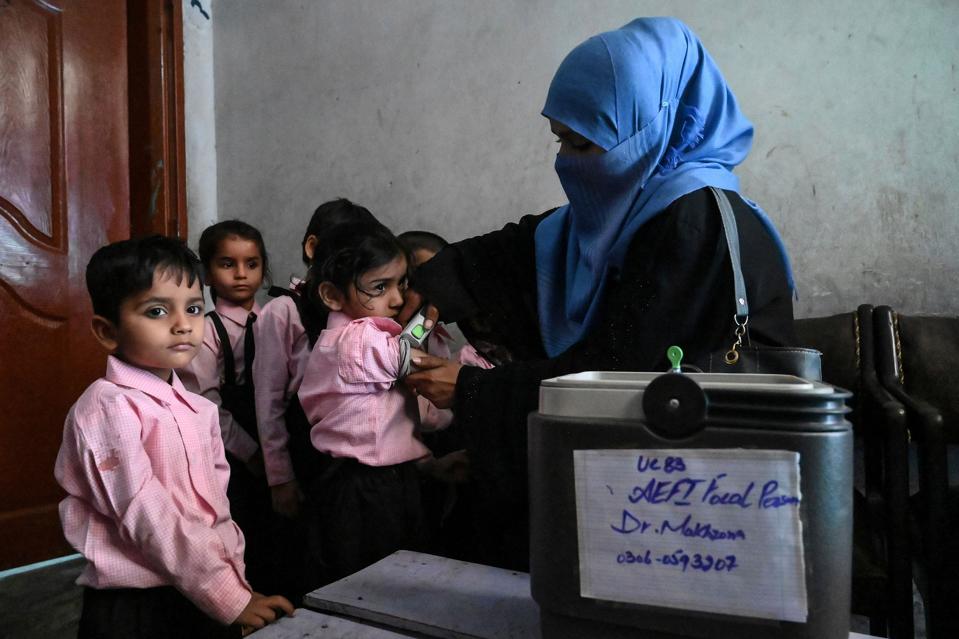
A health worker administers Inactivated polio vaccine (IPV) to a child during a polio vaccination campaign at a school in Lahore on March 2, 2021. (Photo by Arif ALI / AFP) (Photo by ARIF ALI/AFP via Getty Images)
AFP VIA GETTY IMAGES
Though we’ve cycled through many kinds of public health interventions in our struggle to stop Covid-19 from spreading, mass vaccination is now the primary means through which we’re aiming to end the pandemic for good. Given this, more than ever we need to be studying mass vaccination campaigns that proved successful. The most astounding of these by far was polio—an eradication effort more than 70 years in the making that, thanks to a new vaccine model, may finally come to an end. In this article I’ll explore the research behind this vaccine in greater detail, not just to elaborate on why it is so impressive, but to delve deeper into its lessons for the current crisis.
Of all the diseases to cause global pandemics in the past century, the only we’ve eradicated so far is polio. Thanks to vaccines, polio prevalence has been reduced to a small sliver of what it once was—at its worst in the late 1940s, infecting hundreds of thousands and paralyzing more than 35,000 people a year. Unlike SARS-CoV-2, the virus that causes Covid-19, the poliovirus exists only in humans, meaning it can’t come at us sideways from an animal reservoir. Even so, parallels exist between the two that are worth considering as our conversations begin to shift from short-term to long-term immunity.
One lesson from polio we must take into account is the importance of distinguishing between a vaccine that prevents disease and a vaccine that prevents transmission. The struggle to eradicate polio drove home the fact that different vaccines elicit different types of immunity, each with its own set of implications for public health. The current generation of Covid-19 vaccines, created by the likes of Moderna and Pfizer, prevents serious illness and death, as evidenced by their enormous success in clinical trials. But whether they will stop transmission is currently unknown.
The distinction is an important one, in some cases a matter of life or death. The inactivated polio vaccine created by Jonas Salk in 1953 is able to prevent disease—that is, the onset of debilitating symptoms like paralysis—and trigger a long-lasting antibody response that can remain in effect for many, many years. But it doesn’t prevent infection or transmission through the nose, mouth, or gut, which is why it wasn’t effective at wiping out the poliovirus altogether. People vaccinated with it could still shed infectious virus. It was ultimately the live-attenuated oral polio vaccine, an invention of Albert Sabin’s used for the first time in 1961, that made the largest contribution to global eradication, not just because it was cheaper and easier to administer, but also by virtue of its ability to prevent the holy trinity of infection, transmission, and disease. How it elicits such powerful immunity remains somewhat a mystery, but its efficacy cannot be denied.
Another important distinction exists between SARS-CoV-2 and poliovirus that is equally instructive. For both viruses the roots of transmission are similar but different. For polio, it’s mostly gut-based but also nasopharyngeal; for Covid-19, it’s the opposite, mostly through the nose and mouth but occasionally the gut, characteristic of respiratory viruses in general. That we still need an annual flu shot is proof of how difficult it has been to develop vaccines capable of engendering long-lasting immunity against respiratory viruses. One reason why is we simply don’t know enough about their immunology more broadly—a gap in our knowledge we’ve had opportunities to fill, but whether for lack of interest, funds, or infrastructure never did.
Intimate knowledge of viral mechanisms of transmission doesn’t come easy or cheap. Our near-global eradication of polio, as well as our deep understanding of how the poliovirus works, is the product of many decades of research dedicated not just to polio specifically but molecular biology, gene manipulation, and advancing biomedicine at large. Funding was in abundance, and the collective will to push the frontiers of what we knew was strong. Over a span of decades scientists around the world studied the virus rigorously, discovering that the main obstacle to permanent eradication was its ability to occasionally regain virulence despite vaccination—hence the handful of cases each year. Every so often, they discovered, the attenuated virus—that is, a non-virulent version—used to create the oral vaccine reverts to its original disease-causing and potentially paralyzing form. Infections caused by these viruses, which are estimated to occur at a rate of 4.7 per million births, are referred to as vaccine associated paralytic poliomyelitis. Though the inactivated virus used in Salk’s vaccine doesn’t cause the same problem, the oral vaccine is so much easier to distribute that merely switching from one to the other isn’t the simple solution it may seem to be.
For decades, vaccine associated paralytic poliomyelitis was considered a necessary risk. But as we’re now well aware, thanks to our struggle to contain Covid-19, so long as one person is infected, no one is totally safe from harm. The good news is that advances in genetic technology are helping us tackle the challenge of viral evolution with greater precision—which is exactly what a group of scientists working under the auspices of the New Oral Poliovirus Vaccine Consortium has done, according to a study published last year in Cell Host & Microbe. By identifying the exact mutations that allow an attenuated poliovirus to become dangerous once again and engineering a version that averts them, this research brings us one step closer to a vaccine that could eradicate polio once and for all.
Perhaps the main reason why the oral polio vaccine is so effective at preventing infection and transmission is its ability to engender immunity in both the mouth, nose, and gut. As I mentioned before, excretions from both these bodily points of entry and exit are the most efficient routes a poliovirus can take—aided and abetted by poor sanitary conditions—to get from one host to another. By introducing an attenuated virus at the intestinal level, the oral vaccine eliminates these modes of contagion, and by extension its ability to circulate freely in the wild.
One of the biggest determinants of attenuation is a point mutation, 481A, in a noncoding region of the poliovirus known as domain V, or domV, that destabilizes the virus’s translation mechanism, in essence keeping it from expressing more lethal forms of itself. It is in the best interest of the virus, however, to mutate and select for structural stability. And when the difference between the two is only a matter of a single nucleotide, it should come as no surprise that in the few VAPP outbreaks that have occurred in recent years, a 481 A-to-G mutation appeared with high frequency.
The goal of the researchers, then, was to engineer a virus that greatly reduced the likelihood of mutation and recombination events—in other words, curtail its capacity to adapt even further—without compromising the process of viral replication that is so essential to building gut immunity. How they did this came down to a total of five modifications, divided up between three areas of focus.
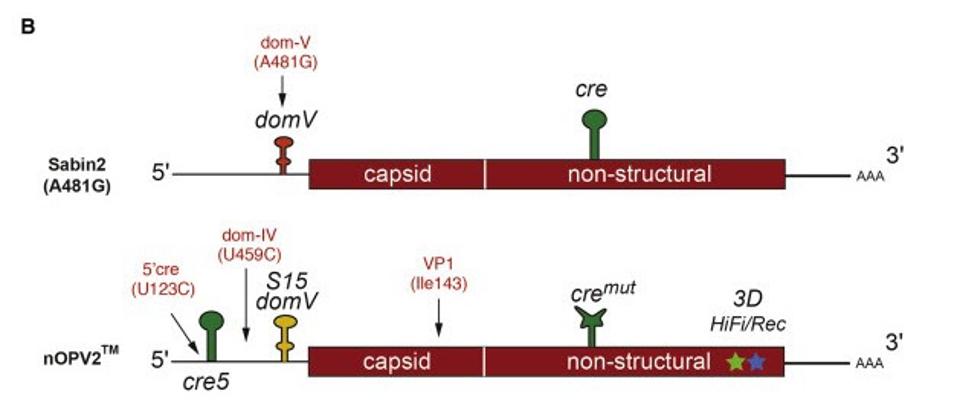
Figure 1. Positions for the most frequent mutations identified from shed viruses: domV A481G in Sabin2 (Evans et al., 1985); U123C (5′ cre), U459C (domIV), and A2969G (VP1-I143V) in nOPV2.
SOURCE: “ENGINEERING THE LIVE-ATTENUATED POLIO VACCINE TO PREVENT REVERSION TO VIRULENCE” HTTPS://WWW.CELL.COM/CELL-HOST-MICROBE/FULLTEXT/S1931-3128(20)30230-4?_RETURNURL=HTTPS%3A%2F%2FLINKINGHUB.ELSEVIER.COM%2FRETRIEVE%2FPII%2FS1931312820302304%3FSHOWALL%3DTRUE#ARTICLEINFORMATION
First was domV, which they not only stabilized but locked into a tight configuration, so as to occlude any possibility of reversion. Second, they altered the error-prone polymerase, an enzyme key to viral evolution and genetic diversity more generally. Last but not least, they relocated a cis-acting replication element (cre) closer to and upstream of domV. That way if the virus does attempt recombination, it won’t be able to swap out the stabilized bits so readily. These alterations, deduced based on evidence from cell culture and animal models, work against reversion in tandem, but are at their core functionally independent, coming together to form a “multilayer safety-net.” Even if mutations still developed in all three of these targeted areas—a highly unlikely occurrence, but minutely possible nonetheless—that strain would still be at least 250 times as attenuated as a reverted version of the current oral vaccine.
The next step was to test the safety and efficacy of the engineered virus through a phase I vaccination trial. To work, the new vaccine, which the researchers called nOPV2, had to be just as antigenic and immunogenic as before, but without the near omnipresence of reversion-enabling mutations in the shed virus of trial participants. Strikingly, trial participants who received a dose of the nOPV2 vaccine had no mutations, including at the 481 site, in the domV region, confirming that the modifications had successfully impeded viral evolution without affecting replication.
What these researchers were able to achieve by using new technology to rigorously analyze the poliovirus, its enabling mechanisms, and countermeasures against it is nothing short of remarkable. Therein lies the final and arguably most important lesson that polio research has for those of us trying to unravel the current crisis. To create effective, long-lasting countermeasures like vaccines that will grant us immunity from Covid-19 not just this year, but the next and the many thereafter, we need to understand the virus SARS-CoV-2 in much greater detail, pouring as much funding and resources into coronavirus research as we did for polio back in the 1940’s and 50’s. Such an effort would come with the added bonus of shedding light on other respiratory viruses—primarily influenza—that have mystified us for so long.
In my next piece for this series, I will delve further into how the battle against polio was won, focusing specifically on a factor that isn’t altogether missing from efforts to stop the current pandemic, but could certainly be improved: global cooperation.
Originally published on Forbes (March 8, 2021)