Understanding Omicron: A Structure-Function Tour De Force
(Posted on Friday, January 28, 2022)
This is the second in a series on the Omicron variants.
Scientific progress in understanding Omicron has been remarkably swift since its discovery in late November of last year. The newest paper from the University of Washington by McCallum et al. is a tour de force in helping us understand the properties of Omicron in molecular detail. It is a marvel of modern science that such depth and understanding of a new form of a dangerous pathogen can be unraveled in such a short time. Just two weeks ago, we wrote our first story about the structural-functional relationship of the genome and proteins of the Omicron variant infecting the United States and most of Europe at that time.
In previous weeks, there was little appreciation of the potential importance not only of the predominant form of the Omicron variant, now called BA.1 but of its sibling variants—BA.2 and BA.3—that arose simultaneously and are now a topic of great interest as BA.2 is on the rise. This series discusses what’s known about these three variants in more detail.
McCallum and colleagues employed two techniques to understand the structural-functional relationship of mutations in the Spike protein of BA.1: cryo-electron microscopy and X-ray crystallography. Both of these techniques can elucidate the structure of proteins at near-atomic resolution.
ACE2 Binding
One of the outstanding questions of Omicron is why it is so much more transmissible than the original Wuhan strain or any of the following variants: Alpha, Beta, Gamma, and Delta. The initial thought is that the properties of the Spike protein, particularly the affinity of binding to the ACE2, would reveal the answer. McCallum et al.’s work reflects what other studies have found. Omicron binds the ACE2 receptor less tightly than many previous variants and only about two and a half times as tightly as the Wuhan strain. Molecular analysis of the Spike protein reveals why this is the case.
Some mutations, like lysine to asparagine at position 417 (K417N), glutamine to arginine at position 493 (Q493R), and glutamine to arginine at position 498 (Q498R), are predicted to reduce the binding affinity to ACE2 by modifying the salt bridges in the Spike. Others, like asparagine to tyrosine at position 501 (N501Y) and serine to asparagine at position 477 (S477N), have been shown to increase ACE2 affinity by creating new hydrogen bonds. Collectively, these changes add up to no more than a 2.4-fold increase in binding affinity relative to the Wuhan isolates.
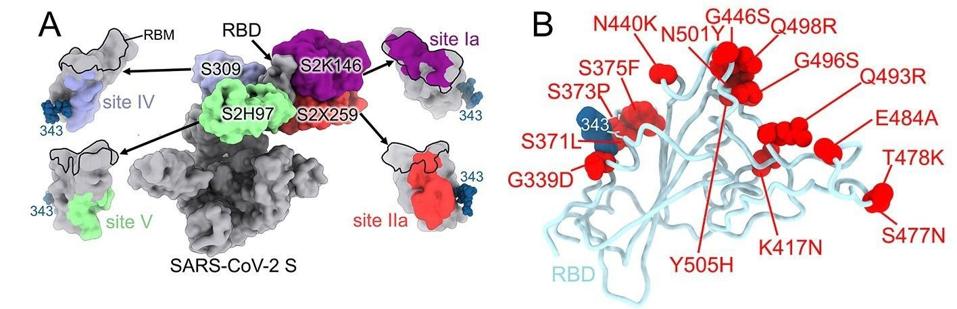
FIGURE 1: SARS-CoV-2 Omicron RBD mutations promote escape from a panel of clinical mAbs. (A) RBD antigenic map as determined elsewhere. (B) Ribbon diagram of the RBD crystal structure with residue mutated relative to the Wuhan-Hu-1 RBD shown as red
MCCALLUM ET AL.
In conclusion, the Omicron receptor-binding domain affinity does not fully account for the variant’s increased infectivity. For example, the Beta variant binds to the ACE2 receptor more tightly than Omicron, but it is substantially less transmissible.
Immune Evasion
The ability of Omicron to evade the immune response, elicited either by natural infection or by vaccination, is now legendary. Although people who have recently been triple vaccinated have some protection against Omicron infection, the protection from natural or vaccine-induced antibodies fades to close to zero between three and five months. It is essential to distinguish between protection from transmission and serious disease. Most, if not all, vaccines provide substantial protection, in some cases up to 90% or greater, from hospitalization, serious illness, and death for a year or more.
The study provides a detailed explanation at an atomic resolution of how viruses evade natural immune defenses and monoclonal antibodies in an elegant atom by atom tour. The work also describes why at least one FDA-approved monoclonal antibody, sotrovimab, maintains much of its activity.
McCallum and colleagues describe each of the mutations and how they contribute to escape from monoclonal antibodies that are effective against other variants. They suggest that amino acid mutations threonine to isoleucine at position 95 (T95I), deletion at position 211 (del211), leucine to isoleucine at position 212 (L212I), and insertion of glutamic acid-proline-glutamic acid at position 214 (ins214EPE) modulate recognition by some of the antibodies, specifically S2L20 and S2X333.
Glycine to aspartic acid at position 142 (G142D) and deletion of positions 143 to 145 (del143-145) account for evasion from neutralization mediated by antibodies that recognize the N-terminal domain specifically.
Amino acid substitutions lysine to asparagine at position 417 (K417N), glycine to serine at position 466 (G446S), serine to asparagine at position 477 (S477N), threonine to lysine at position 478 (T478K), glutamic acid to alanine at position 484 (E484A), glutamine to arginine at position 493 (Q493R), glycine to serine at position 496 (G496S), glutamine to arginine at position 498 (Q498R), and tyrosine to histidine at position 505 (Y505H) are all shown to inhibit at least one binding site from a monoclonal antibody (Figure 1B). These mutations use a combination of steric hindrance, electrostatic contact remodeling, abrogating electrostatic interactions, and so on. These and the rest of the N-terminal domain and receptor-binding domain mutations work similarly to reduce the binding of convalescent and vaccine antibodies.
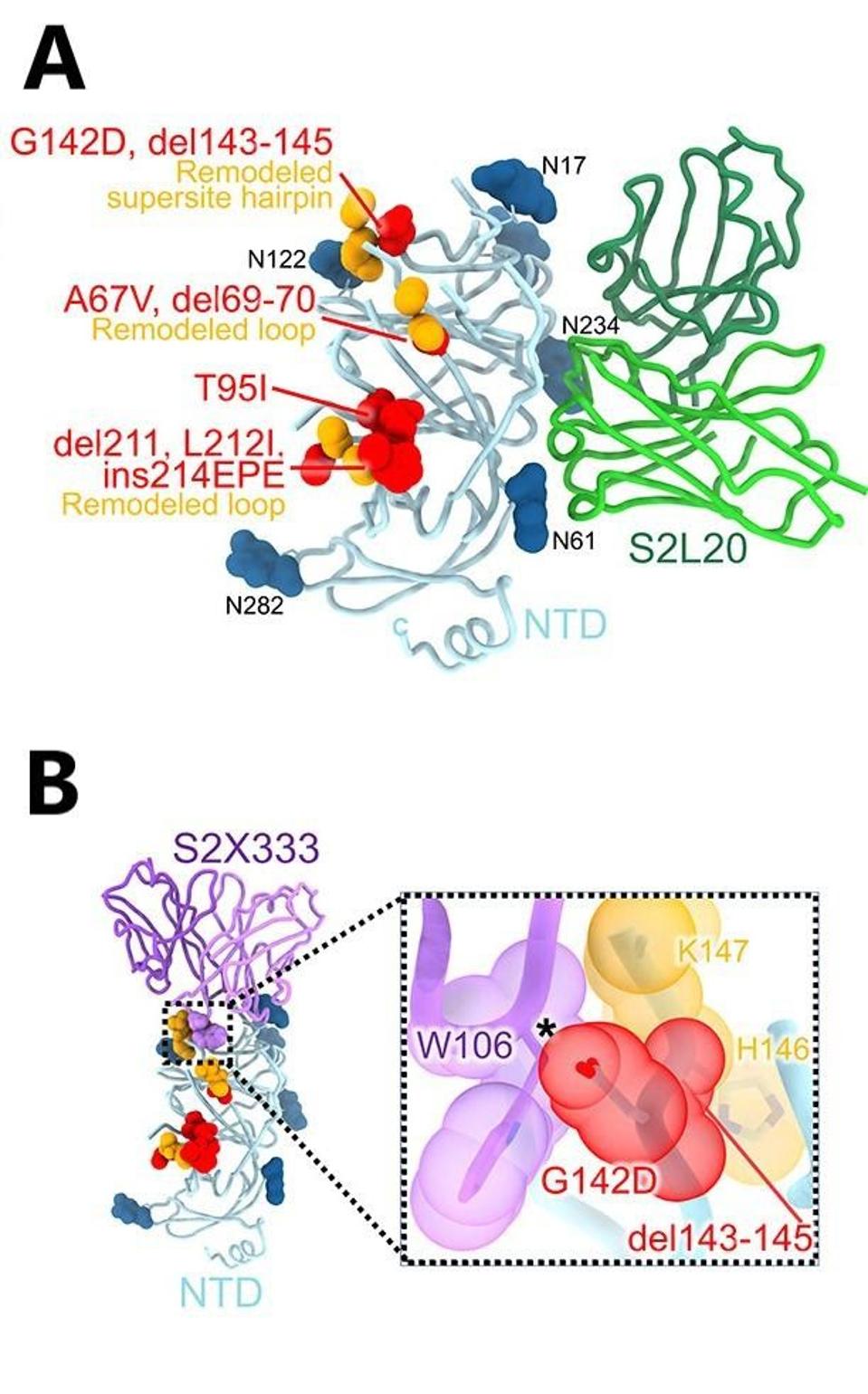
FIGURE 2: (A) S2L20-bound Omicron NTD with mutated, deleted, or inserted residues rendered or indicated as red spheres. Segments with notable structural changes are shown in orange and labeled. (B) Omicron NTD overlaid with the S2X333 Fab,
MCCALLUM ET AL.
In conclusion, the molecular analysis provides a very firm basis for understanding the ability of Omicron to view evade both natural, vaccine-induced natural, and monoclonal antibody neutralization.
Endosomal Entry
Another prominent feature, which has come as a great surprise to those who study the virus, is that the route of entry for the Omicron family of viruses is remarkably different from that of previous strains. Those viruses enter membrane-to-membrane, which occurs after binding. Two proteolytic cleavage events release the fusion peptide, forming a membrane bridge between the virus and the cell. This allows the virus nucleic acids to enter the cell and begin replicating.
Such is not the case for the Omicron family, which enters via the endosomal pathway. The whole virus enters the cell in a bubble instead of membrane entry. Once within the bubble, the virus can break through and spread.
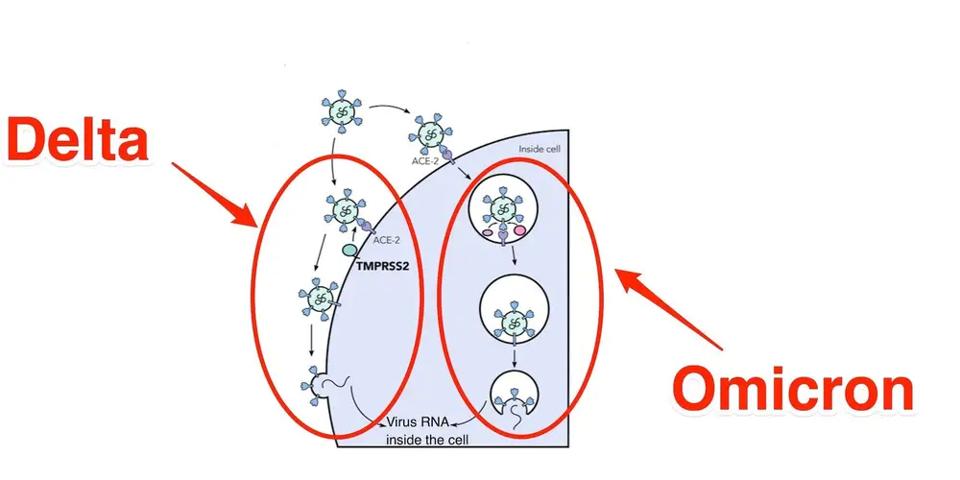
FIGURE 3: Membrane to membrane vs. endosomal entry of SARS-CoV-2 in Delta vs. Omicron
GEORGINA BROWN/JOE GROVE/INSIDER
Why does this occur? Mutations near the furin cleavage site in many variants led some to believe they would enhance cleavage efficiency. In the Omicron variants, there are many more mutations in these regions. However, McCallum et al. show that some of these mutations in the Omicron Spike protein interfere with cleavage, reducing efficiency.
The mutations in this region reduce fusion and cleavage efficiency by introducing inter-promoter electrostatic contacts, improving intra-promoter hydrophobic packing, and so on. The mutations, threonine to lysine at position 547 (T547K), histidine to tyrosine at position 655 (H655Y), asparagine to lysine at position 764 (N764K), aspartic acid t tyrosine at position 796 (D796Y), histidine to lysine at position 856 (N856K), glutamine to histidine at position 954 (Q954H), asparagine to lysine at position 969 (N969K), and leucine to phenylalanine at position 981 (L981F), may work synergistically to yield reductions in these processes.
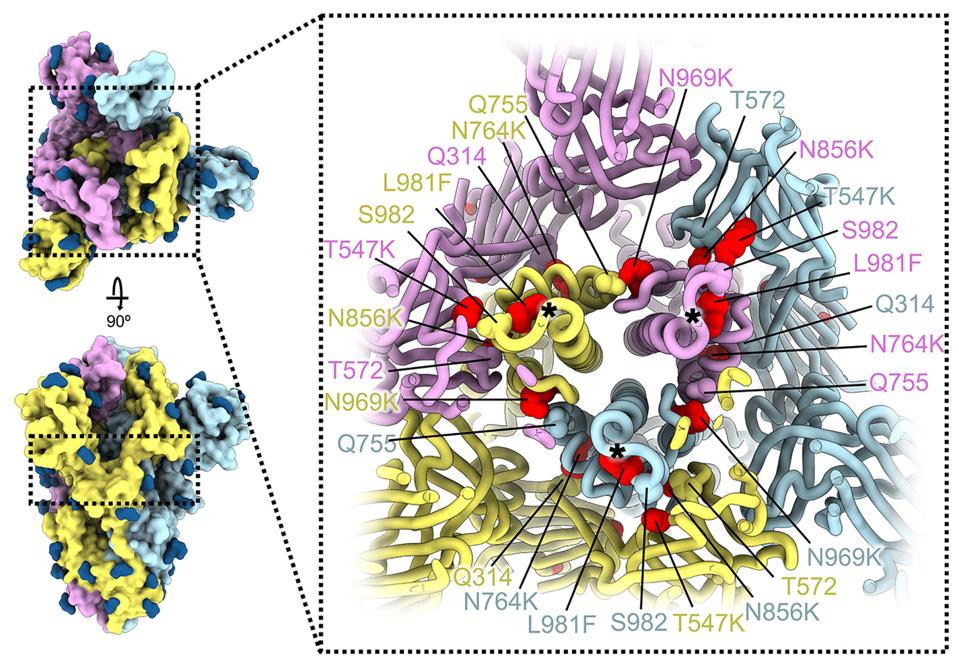
FIGURE 4: SARS-CoV-2 Omicron S mutations outside the NTD and RBD.
MCCALLUM ET AL.
This work is a dramatic advance in our knowledge of the details of Omicron. McCallum et al. elucidate some of its most outstanding characteristics, differentiating from its predecessor variants of concern. However, many mysteries remain. Why this virus is so much more transmissible does not seem to be a property solely of the Spike protein. We note that several other mutations in the other proteins in the genome and silent mutations may alter regulatory sequences, potentially accounting for increased transmissibility.
This leads to an interesting comparison over time between Omicron and Delta. A recent study by Hay et al. noted that Delta infections yield a greater viral load than Omicron, and infection durations are typically longer in Delta. However, Omicron’s incubation period is shorter than Delta by roughly a day, meaning after initial infection, a host with Omicron is infectious to others faster than Delta.
Absence of Cell-to-cell Fusion
Another mystery is Omicron’s reduced syncytia formation. Once a cell is infected with SARS-CoV-2 or some other dangerous pathogens, it can fuse with neighboring cells in an evet called syncytia formation. Therefore, syncytia formation is typically associated with pathogenicity. However, the mutations in the Spike protein, particularly those in the furin and fusion regions, lead to reduced fusogenicity, meaning fusion is less efficient. This is likely what leads to the endosomatic entry. But how this virus came to develop these attributes, we do not know, as most variants to this point of the pandemic have continued to grow in pathogenicity, not reduce.
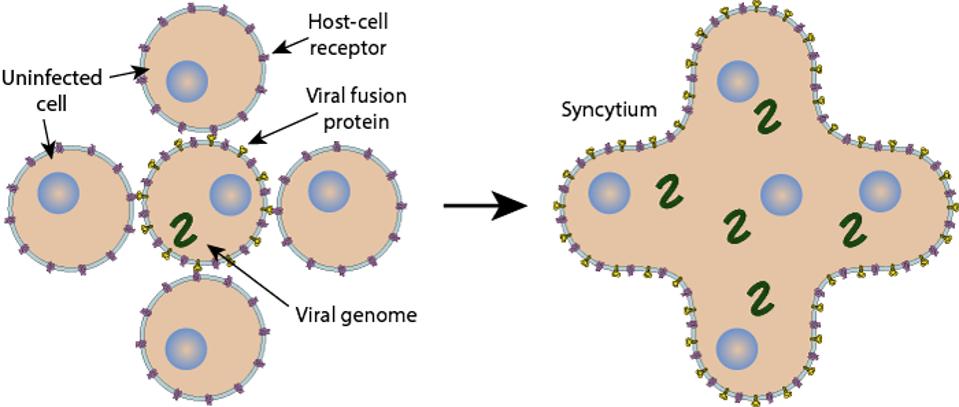
FIGURE 5: Syncytia formation.
VIRAL ZONE
The Virulence Mystery
A third mystery is why this virus is less likely to induce hospitalization and death. Some speculate that the requirement for endosomal entry and the absence of cell-to-cell fusion may be part of replicating preferentially in the nasopharynx instead of the deep lung. The speculation suggests that the requirement for endosomal entry is one reason omicron is less pathogenic. Although there may be some merit to the argument, recall that SARS-CoV, the virus responsible for the SARS epidemic, also enters cells via the endosome, not by fusion. SARS-CoV is highly pathogenic, killing about 4% of young adults and 45% of those over 65. The deep lung pathology of SARS resembles that of Covid-19. I doubt that the difference in the pathology of Omicron virus earlier variants of SARS-CoV-2 is due solely to differences in the spike protein.
We have much more to learn about the function of the exterior glycoproteins and the 29 other proteins of SARS-CoV-2.