How mRNA Could Cure Sickle Cell Gene Mutations
(Posted on Wednesday, August 23, 2023)
Originally published on Forbes on August 18, 2023.
This story is part of a series on the current progression in Regenerative Medicine. In 1999, I defined regenerative medicine as the collection of interventions that restore to normal function tissues and organs that have been damaged by disease, injured by trauma, or worn by time. I include a full spectrum of chemical, gene, and protein-based medicines, cell-based therapies, and biomechanical interventions that achieve that goal.
This piece discusses advances in stem cell gene therapy via mRNA technology. Part One describes how mRNA could reinvent how we prepare patients for bone marrow transplants. The final installment will discuss how to alter blood stem cells with the same system.
Sickle cell disease is the most common genetic blood disorder in the United States and affects 100,000 Americans. A bone marrow or blood stem cell transplantation remains the only curative therapeutic option, but it can be challenging to qualify for the treatment or risky to undergo. Researchers at the University of Pennsylvania investigated a new, potentially less toxic method that corrects the pathogenic genes at their source. The promising preclinical results build anticipation for an enhanced future treatment to come.
Treating Sickle Cell Disease
Sickle cell disease encompasses several inherited blood disorders that arise from mutations in a protein called hemoglobin. Hemoglobin is essential for red blood cell function. Oxygen attaches to this protein inside red blood cells and then is carried throughout the body.
People with sickle cell disease inherit a gene from each parent that encodes for irregular hemoglobin. Abnormal hemoglobin deforms the flexible, round shape red blood cells typically have. The cells turn rigid, sticky and crescent or sickle-shaped. In turn, the blood cells more readily clump together and block blood flow. The resulting lack of oxygen to vital organs and tissues manifests complications such as infection and stroke.
The Cost of a Cure
This lifelong condition can only be cured through a bone marrow or blood stem cell transplant. Sickle red blood cells develop from hematopoietic stem cells that carry the mutated hemoglobin gene. The procedure rids the patient of these diseased blood stem cells and replaces them with healthy ones, allowing the body to produce healthy red blood cells.
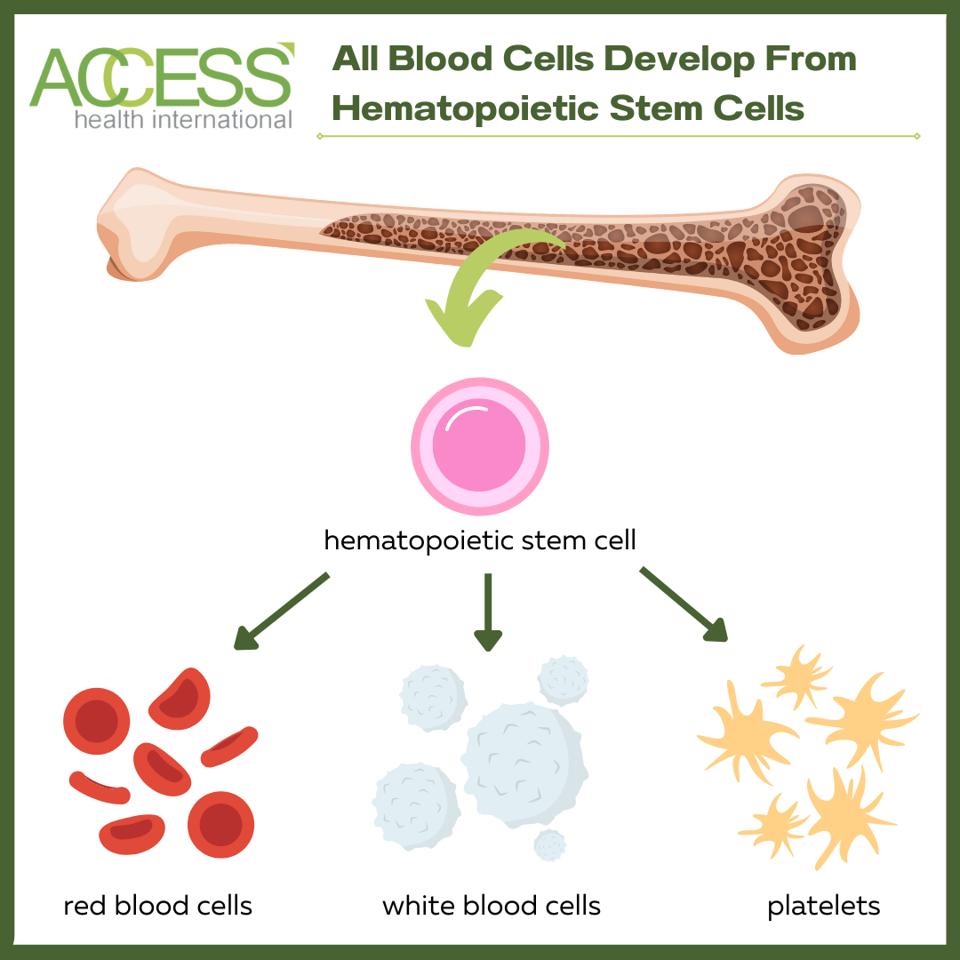
Figure 1: All blood cells originate and develop from hematopoietic stem cells. Hematopoietic stem cell transplantation replaces abnormal, disease-causing hematopoietic stem cells with healthy ones to replenish the blood.
ACCESS HEALTH INTERNATIONAL
This permanent solution may sound tempting, but few can withstand the possible hazards. As with other organ transplants, the body may reject the donated cells—even if they originate from close relatives—and stir up potentially life-threatening immune responses. Another alternative is to source the cells from the patient and correct the genes in the lab. In either case, the patient cannot avoid the toxicities of chemotherapy or radiation used to prime the bone marrow before transplantation.
Correcting Gene Mutations with mRNA
Is it possible to cure sickle cell disease without the high stakes? Researchers at the University of Pennsylvania probed an innovative avenue: correcting the genetic mutation at its source with mRNA technology.
mRNA technology depends on lipid nanoparticles to deliver genetic information to a programmed target—in this case, pathogenic hematopoietic stem cells. As discussed in a previous article, the team decorated the lipid nanoparticles with an antibody that detects a protein called antigen CD117 on the surface of blood stem cells. After the blood stem cell encircles the lipid nanoparticle, the nanoparticle breaks down and releases the desired mRNA. The cell then reads the mRNA and produces proteins based on its instructions, thus activating a cellular response.
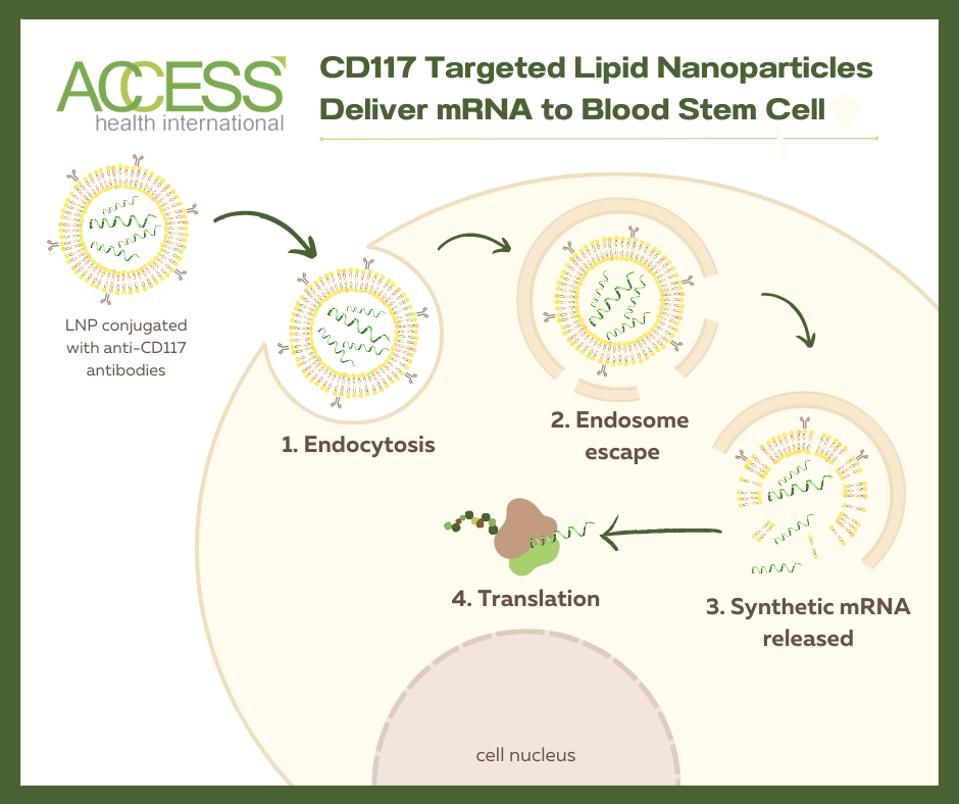
Figure 2: mRNA technology relies on antibody-decorated lipid nanoparticles to deliver the desired mRNA to the target cell (here, a hematopoietic stem cell). The target cell engulfs the nanoparticle, creating a pocket-like space called an endosome. Next, the endosome begins to break down, exposing the nanoparticle to degradation. This frees the desired mRNA into the cell cytoplasm, where it can be translated to create proteins necessary to activate apoptosis.
ACCESS HEALTH INTERNATIONAL
In this experiment, the anti-CD117 lipid nanoparticles contained either: 1) gene-editing mRNA that encodes a Cas9 adenine base editor fusion protein or 2) a single-guide RNA that should direct the mRNA to the hemoglobin sickle cell mutation.
Cas9 is a recognizable component of the CRISPR-Cas9 gene editing system. Here, the protein converts a single DNA base (adenine) to another base (guanine). The A to G conversion turns pathogenic hemoglobin DNA into a harmless, nonpathogenic variant. The nonpathogenic variant is not the typical hemoglobin seen in non-sickle cell patients; even though this variant does not naturally occur in humans, it is still functional.
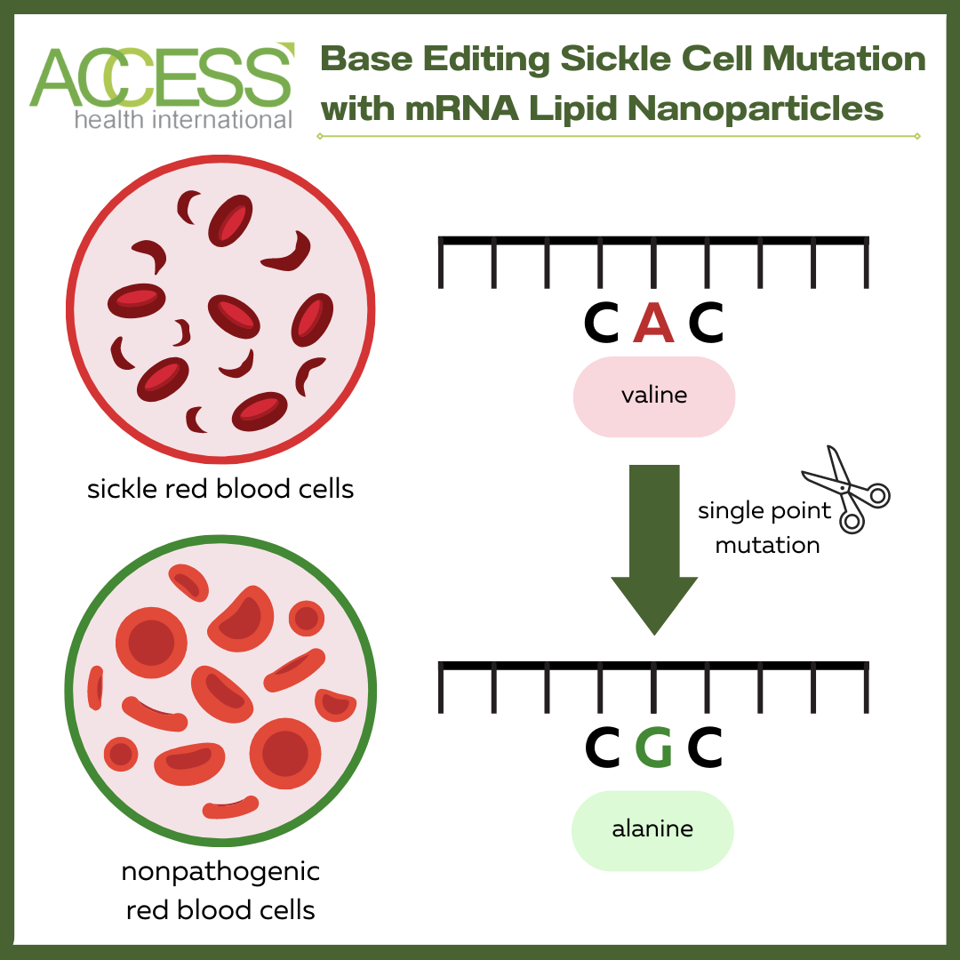
Figure 3: In this study, the Cas9 adenine base editor fusion protein, along with the single-guide RNA, target and convert pathogenic hemoglobin DNA into a harmless variant. The base editor specifically converts an adenine:thymine (A:T) base pair into a guanine:cytosine (C:T) base pair. The change produces a different protein, alanine instead of valine, and turns the sickle cell hemoglobin into a nonpathogenic version.
ACCESS HEALTH INTERNATIONAL
Four sickle cell specimens from separate donors simultaneously received the two types of nanoparticles. The researchers then observed the cell cultures, noting the number of pathogenic and nonpathogenic hemoglobins to see if the DNA was successfully corrected. Applying an excess of guide RNA to mRNA led to efficient base editing. In these cases, nonpathogenic hemoglobin numbers increase by more than 90%; comparatively, pathogenic hemoglobin decreases.
The cell cultures were also exposed to hypoxic conditions, mimicking the low-oxygen environments sickle cells create when blocking the blood vessels. The nonpathogenic hemoglobin should behave normally and resist sickling red blood cells if adequately corrected.
The team found that editing levels and the increase in nonpathogenic hemoglobin were directly correlated. In addition, even the highest dose of lipid nanoparticles did not inhibit the growth and function of the blood stem cells. The results combined suggest that base-editing mRNA, when paired with single-guide RNA, can neutralize the threat of sickle red blood cell mutations.
Looking Forward
mRNA’s potential applications continue to expand. Not only can mRNA-carrying lipid nanoparticles deplete stem cells, they can also accurately edit the human genome as demonstrated in this study. Although undoubtedly in its early stages, this finding holds immense therapeutic potential if clinically translated. A single injection of mRNA lipid nanoparticles could cure patients with sickle cell diseases—a potentially safer and simpler alternative to bone marrow transplants.
The final installation of this mRNA series will explore another developing use for mRNA: editing hematopoietic stem cells in vivo.
To read more of this series, please visit www.williamhaseltine.com